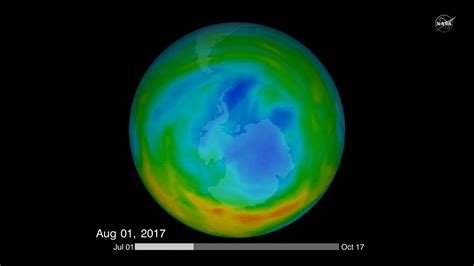
The Antarctic ozone hole, discovered four decades ago, shows signs of recovery thanks to international efforts to ban ozone-depleting substances, but its complete healing remains uncertain and faces new threats from climate change and unregulated chemicals.
The discovery of the ozone hole in 1985 shocked the world, revealing a severe thinning of the ozone layer over Antarctica. This protective layer, vital for shielding life on Earth from harmful ultraviolet (UV) radiation, was being depleted by human-produced chemicals, primarily chlorofluorocarbons (CFCs) used in refrigerants, aerosols, and other industrial applications. Scientists like Joe Farman, Brian Gardiner, and Jonathan Shanklin from the British Antarctic Survey were the first to identify this dramatic loss, sparking global alarm and leading to unprecedented international cooperation. Their work highlighted a stark contrast between the “normal ozone values of around 300 DU (Dobson Units) and the shockingly low 200 DU, or even 150 DU, that they were seeing.”
The Montreal Protocol, ratified in 1987, stands as a landmark achievement in environmental diplomacy. This international treaty committed nations to phasing out the production and consumption of ozone-depleting substances. “The Montreal Protocol proves that when the world works together, we can solve seemingly insurmountable environmental problems,” said Inger Andersen, Executive Director of the UN Environment Programme, underscoring the agreement’s monumental success.
While the Montreal Protocol has been largely successful, recent scientific findings suggest that the ozone layer’s recovery is not guaranteed. The ozone hole still forms annually over Antarctica, and its size and severity are influenced by meteorological conditions. Furthermore, the changing climate is altering atmospheric circulation patterns, which could affect the distribution of ozone in the stratosphere. The complete recovery of the ozone layer to pre-1980 levels is now projected to occur around 2066, later than previous estimates.
Emerging threats also complicate the ozone layer’s recovery. Unregulated very short-lived substances (VSLS), used as industrial solvents and paint strippers, are not controlled by the Montreal Protocol. Although they have short atmospheric lifetimes, their increasing use could contribute to ozone depletion, particularly in certain regions. Furthermore, geoengineering proposals, such as stratospheric aerosol injection to combat climate change, could have unintended consequences for the ozone layer.
Discovery and Initial Impact
In 1985, the scientific community was rocked by the discovery of a massive hole in the ozone layer above Antarctica. This depletion, far exceeding any previously anticipated levels, was documented by British Antarctic Survey scientists Joe Farman, Brian Gardiner, and Jonathan Shanklin. Their research revealed that the ozone layer, which normally absorbs harmful ultraviolet (UV) radiation from the sun, had thinned dramatically during the Antarctic spring. This thinning allowed significantly more UV radiation to reach the Earth’s surface, posing a serious threat to human health and ecosystems.
The scientists’ findings were particularly alarming because they contradicted existing models of atmospheric chemistry. These models had not predicted such a rapid and severe decline in ozone levels. The initial reaction from some members of the scientific community was skepticism, but Farman and his team meticulously verified their data, demonstrating the reality of the ozone hole. The discovery triggered a flurry of research aimed at understanding the causes and consequences of ozone depletion.
The ozone layer, located in the stratosphere approximately 15 to 30 kilometers above the Earth’s surface, is crucial for protecting life on Earth. Ozone molecules (O3) absorb UV radiation, preventing it from reaching the ground. UV radiation is known to cause skin cancer, cataracts, and immune system suppression in humans. It can also damage plants, marine organisms, and other components of the ecosystem.
The Culprits: Ozone-Depleting Substances
The scientific investigation that followed the discovery of the ozone hole quickly identified the culprits: ozone-depleting substances (ODS), particularly chlorofluorocarbons (CFCs), halons, and other industrial chemicals. CFCs, widely used as refrigerants, aerosol propellants, and solvents, were found to be particularly damaging. These chemicals are very stable and can persist in the atmosphere for decades.
When CFCs reach the stratosphere, they are broken down by UV radiation, releasing chlorine atoms. These chlorine atoms act as catalysts in a chain reaction that destroys ozone molecules. A single chlorine atom can destroy thousands of ozone molecules before it is eventually removed from the stratosphere. The process is particularly efficient in the cold, stable conditions of the Antarctic stratosphere during the spring, leading to the formation of the ozone hole.
Halons, used in fire extinguishers, contain bromine atoms, which are even more effective at destroying ozone than chlorine. Other ODS, such as methyl bromide (used as a fumigant) and carbon tetrachloride (used as a solvent), also contribute to ozone depletion.
The Montreal Protocol: A Triumph of International Cooperation
The discovery of the ozone hole and the identification of ODS as the cause led to swift and decisive international action. In 1987, the Montreal Protocol on Substances That Deplete the Ozone Layer was adopted. This landmark agreement committed signatory nations to phasing out the production and consumption of ODS.
The Montreal Protocol is widely regarded as one of the most successful environmental treaties in history. It has been ratified by every country in the world and has led to a dramatic reduction in the atmospheric concentrations of ODS. The protocol has been amended several times to accelerate the phase-out of ODS and to include additional chemicals.
The success of the Montreal Protocol is attributed to several factors, including strong scientific evidence linking ODS to ozone depletion, the availability of cost-effective alternatives to ODS, and the willingness of governments, industry, and civil society to work together. The protocol also includes provisions for financial assistance to developing countries to help them comply with its requirements.
Signs of Recovery and Ongoing Challenges
As a result of the Montreal Protocol, the ozone layer is showing signs of recovery. Scientific studies have documented a decrease in the size and severity of the Antarctic ozone hole. The concentration of ODS in the atmosphere is declining, and the ozone layer is projected to return to pre-1980 levels by the middle of the 21st century. A 2018 report noted the hole would recover “by 2060s”.
However, the recovery of the ozone layer is not guaranteed. Several challenges remain.
- Long Atmospheric Lifetimes of ODS: Many ODS have long atmospheric lifetimes, meaning that they will continue to deplete the ozone layer for decades to come.
- Illegal Production and Consumption of ODS: Despite the Montreal Protocol, there have been instances of illegal production and consumption of ODS, particularly in developing countries.
- Climate Change: Climate change is altering atmospheric conditions, which could affect the rate of ozone recovery. Changes in temperature, atmospheric circulation, and cloud cover could all influence ozone depletion.
- Emerging Threats: New chemicals and technologies could pose a threat to the ozone layer. For example, some geoengineering proposals involve injecting aerosols into the stratosphere, which could have unintended consequences for ozone depletion. Very short-lived substances (VSLS), not controlled by the Montreal Protocol, are also a growing concern.
The Impact of Climate Change on Ozone Recovery
Climate change is inextricably linked to the recovery of the ozone layer. While the Montreal Protocol focused on phasing out ODS, climate change presents a different set of challenges that could either hinder or accelerate ozone recovery.
One of the most significant impacts of climate change is the alteration of atmospheric temperatures and circulation patterns. As greenhouse gas concentrations increase, the lower atmosphere (troposphere) warms, while the upper atmosphere (stratosphere) cools. This cooling of the stratosphere can exacerbate ozone depletion, particularly in polar regions.
Colder temperatures in the stratosphere promote the formation of polar stratospheric clouds (PSCs), which provide surfaces for chemical reactions that destroy ozone. These reactions are particularly efficient in the presence of chlorine and bromine from ODS. Therefore, as the stratosphere cools due to climate change, it could prolong the period of ozone depletion, even as ODS concentrations decline.
Furthermore, climate change is altering atmospheric circulation patterns, which could affect the distribution of ozone in the stratosphere. Changes in the Brewer-Dobson circulation, a global air circulation pattern that transports ozone from the tropics to the poles, could lead to changes in ozone concentrations in different regions. Some studies suggest that climate change could strengthen the Brewer-Dobson circulation, leading to increased ozone concentrations in the tropics and decreased concentrations in the polar regions.
However, the exact impact of climate change on ozone recovery is still uncertain. Complex interactions between temperature, circulation, and chemical processes make it difficult to predict the future evolution of the ozone layer.
Unregulated Substances: A New Frontier
While the Montreal Protocol successfully addressed the issue of long-lived ODS, it did not regulate very short-lived substances (VSLS). VSLS have atmospheric lifetimes of less than six months, meaning that they are quickly removed from the atmosphere. However, their increasing use as industrial solvents and paint strippers is raising concerns about their potential impact on the ozone layer.
VSLS, such as dichloromethane, can reach the stratosphere in sufficient quantities to contribute to ozone depletion, particularly in certain regions. Their short lifetimes mean that their impact is more localized, but their increasing emissions could offset some of the gains made by the Montreal Protocol.
The scientific community is actively researching the impact of VSLS on the ozone layer. Studies have shown that dichloromethane concentrations in the atmosphere have been increasing rapidly in recent years. Some models predict that VSLS could delay the recovery of the ozone layer by several years.
The regulation of VSLS is a complex issue. Their short lifetimes make it difficult to monitor their emissions and to assess their impact on the ozone layer. Furthermore, VSLS are used in a wide range of applications, making it challenging to find suitable alternatives. However, the growing evidence of their potential impact on the ozone layer suggests that further action may be necessary.
Geoengineering and the Ozone Layer: A Potential Threat
As the world struggles to address climate change, some scientists have proposed geoengineering techniques to mitigate its effects. One such technique is stratospheric aerosol injection (SAI), which involves injecting aerosols into the stratosphere to reflect sunlight back into space and cool the planet.
While SAI could potentially reduce global warming, it could also have unintended consequences for the ozone layer. Some studies have shown that aerosols injected into the stratosphere could enhance ozone depletion by providing surfaces for chemical reactions similar to those that occur on polar stratospheric clouds.
The impact of SAI on the ozone layer would depend on the type and amount of aerosols injected, as well as the location and timing of the injections. Some aerosols, such as sulfate aerosols, are known to be more damaging to the ozone layer than others. Furthermore, the injection of aerosols into the polar regions could exacerbate ozone depletion in those areas.
The potential risks of SAI to the ozone layer highlight the need for careful consideration of the environmental impacts of geoengineering technologies. Before deploying SAI on a large scale, it is essential to conduct thorough research to assess its potential effects on the ozone layer and other aspects of the environment.
Continued Monitoring and Research: Ensuring the Ozone Layer’s Future
The recovery of the ozone layer is a long and complex process that requires continued monitoring and research. Scientists are using a variety of tools, including ground-based instruments, satellite observations, and computer models, to track the evolution of the ozone layer and to understand the factors that influence its recovery.
Ground-based instruments, such as Dobson spectrophotometers, have been used for decades to measure ozone concentrations in the atmosphere. These instruments provide valuable long-term data records that are essential for tracking trends in ozone depletion and recovery.
Satellite observations provide a global view of the ozone layer. Instruments on satellites, such as the Ozone Monitoring Instrument (OMI) and the Aura Microwave Limb Sounder (MLS), measure ozone concentrations and other atmospheric parameters. These observations are used to create ozone maps and to monitor the size and severity of the ozone hole.
Computer models are used to simulate the complex chemical and physical processes that govern ozone depletion and recovery. These models are used to predict the future evolution of the ozone layer and to assess the impact of different factors, such as climate change and VSLS emissions.
Continued monitoring and research are essential for ensuring the complete recovery of the ozone layer. By tracking the evolution of the ozone layer and understanding the factors that influence its recovery, scientists can provide policymakers with the information they need to make informed decisions about protecting the ozone layer.
Conclusion
The ozone hole, once a symbol of environmental crisis, is now showing signs of recovery thanks to the remarkable success of the Montreal Protocol. However, the journey to full recovery is far from over. Climate change, unregulated substances, and potential geoengineering interventions pose new challenges that could delay or even reverse the progress made. Vigilance, continued scientific research, and international cooperation remain crucial to safeguarding the ozone layer and ensuring a healthy planet for future generations. The lessons learned from the ozone crisis offer valuable insights into how the world can address other pressing environmental challenges, such as climate change.
Frequently Asked Questions (FAQ)
-
What exactly is the ozone layer and why is it important?
The ozone layer is a region of Earth’s stratosphere that absorbs most of the Sun’s ultraviolet (UV) radiation. It contains a high concentration of ozone (O3) in relation to other parts of the atmosphere, although still small in relation to other gases in the stratosphere. The ozone layer shields the Earth from harmful UV radiation, which can cause skin cancer, cataracts, immune system suppression, and damage to plant life and marine ecosystems.
-
What caused the ozone hole?
The primary cause of the ozone hole is the release of human-produced chemicals known as ozone-depleting substances (ODS). These substances, including chlorofluorocarbons (CFCs), halons, and other industrial chemicals, were widely used in refrigerants, aerosols, and solvents. When ODS reach the stratosphere, they are broken down by UV radiation, releasing chlorine and bromine atoms that catalytically destroy ozone molecules.
-
What is the Montreal Protocol, and how has it helped the ozone layer?
The Montreal Protocol on Substances That Deplete the Ozone Layer is an international treaty adopted in 1987 that committed signatory nations to phasing out the production and consumption of ODS. It is widely regarded as one of the most successful environmental treaties in history. The Montreal Protocol has led to a dramatic reduction in the atmospheric concentrations of ODS, and the ozone layer is now showing signs of recovery. “The Montreal Protocol proves that when the world works together, we can solve seemingly insurmountable environmental problems,” according to Inger Andersen, Executive Director of the UN Environment Programme.
-
Is the ozone hole completely healed?
No, the ozone hole is not completely healed. While the Montreal Protocol has been largely successful, the ozone layer’s recovery is an ongoing process. The ozone hole still forms annually over Antarctica, and its size and severity are influenced by meteorological conditions. Furthermore, climate change and unregulated substances could delay the recovery of the ozone layer. The complete recovery of the ozone layer to pre-1980 levels is now projected to occur around 2066, later than previous estimates.
-
What are the emerging threats to the ozone layer?
Emerging threats to the ozone layer include:
- Climate Change: Climate change is altering atmospheric conditions, which could affect the rate of ozone recovery.
- Unregulated Substances: Very short-lived substances (VSLS), used as industrial solvents and paint strippers, are not controlled by the Montreal Protocol and could contribute to ozone depletion.
- Geoengineering: Geoengineering proposals, such as stratospheric aerosol injection to combat climate change, could have unintended consequences for the ozone layer.
-
What are very short-lived substances (VSLS) and why are they a concern?
Very short-lived substances (VSLS) are chemicals with atmospheric lifetimes of less than six months. They are used in various industrial applications, such as solvents and paint strippers. While their short lifetimes limit their global impact, increasing emissions of VSLS, particularly dichloromethane, are raising concerns. These substances can reach the stratosphere and contribute to ozone depletion, particularly in certain regions, potentially offsetting some of the gains made by the Montreal Protocol.
-
How could geoengineering techniques, like stratospheric aerosol injection (SAI), affect the ozone layer?
Stratospheric aerosol injection (SAI) is a proposed geoengineering technique that involves injecting aerosols into the stratosphere to reflect sunlight and cool the planet. While SAI could potentially mitigate climate change, it could also have unintended consequences for the ozone layer. Some studies suggest that aerosols injected into the stratosphere could enhance ozone depletion by providing surfaces for chemical reactions similar to those that occur on polar stratospheric clouds. The specific impact depends on the type and amount of aerosols used, as well as the location and timing of injections.
-
What role does the Antarctic play in ozone depletion and recovery?
The Antarctic region is particularly vulnerable to ozone depletion due to its unique meteorological conditions, including extremely cold temperatures and the formation of a polar vortex. These conditions promote the formation of polar stratospheric clouds (PSCs), which facilitate chemical reactions that destroy ozone in the presence of chlorine and bromine from ODS. The Antarctic ozone hole is a severe thinning of the ozone layer that occurs annually during the Antarctic spring. Because of the Montreal Protocol, the ozone hole is showing signs of recovery, but the Antarctic region remains a critical area for monitoring ozone depletion and understanding the factors that influence its recovery.
-
What can individuals do to help protect the ozone layer?
While the primary responsibility for protecting the ozone layer lies with governments and industries, individuals can take several steps to contribute to the effort:
- Support Policies: Advocate for policies that promote the phase-out of ODS and support the Montreal Protocol.
- Proper Disposal: Ensure proper disposal of appliances and equipment that contain ODS, such as refrigerators and air conditioners.
- Reduce Chemical Use: Minimize the use of products that contain harmful chemicals, such as some aerosols and solvents.
- Energy Conservation: Conserve energy to reduce greenhouse gas emissions, which can indirectly affect the ozone layer.
- Stay Informed: Stay informed about the latest research on ozone depletion and climate change, and share this information with others.
-
How are scientists monitoring the ozone layer’s recovery?
Scientists use a variety of tools and techniques to monitor the ozone layer’s recovery:
- Ground-Based Instruments: Instruments like Dobson spectrophotometers provide long-term data records of ozone concentrations in the atmosphere.
- Satellite Observations: Satellites equipped with instruments such as the Ozone Monitoring Instrument (OMI) and the Aura Microwave Limb Sounder (MLS) provide a global view of the ozone layer and measure ozone concentrations.
- Computer Models: Complex computer models simulate the chemical and physical processes that govern ozone depletion and recovery, allowing scientists to predict the future evolution of the ozone layer and assess the impact of different factors.
-
What are the long-term implications if the ozone layer doesn’t fully recover?
If the ozone layer does not fully recover, the long-term implications could be severe:
- Increased UV Radiation: Higher levels of UV radiation reaching the Earth’s surface would lead to increased rates of skin cancer, cataracts, and immune system suppression in humans.
- Ecological Damage: Damage to plants, marine organisms, and other components of the ecosystem could disrupt food chains and reduce biodiversity.
- Climate Change Feedback: Changes in the ozone layer could affect atmospheric temperatures and circulation patterns, potentially exacerbating climate change.
- Economic Impacts: Increased healthcare costs, reduced agricultural productivity, and other economic impacts could strain resources and hinder development.
-
How does the success of the Montreal Protocol inform our approach to addressing climate change?
The Montreal Protocol offers several key lessons for addressing climate change:
- Scientific Consensus: A strong scientific consensus on the causes and consequences of the problem is essential for mobilizing action.
- International Cooperation: International cooperation and legally binding agreements are necessary to achieve meaningful progress.
- Technological Innovation: The development and adoption of cost-effective alternatives to harmful substances are crucial.
- Financial Assistance: Providing financial assistance to developing countries can help ensure their compliance and promote global equity.
- Phased Approach: A phased approach to reducing emissions, with clear targets and timelines, can provide flexibility and encourage innovation.
-
What is the role of the ozone layer in regulating Earth’s climate?
The ozone layer plays a complex role in regulating Earth’s climate. While its primary function is to absorb UV radiation, ozone also absorbs infrared radiation, contributing to the greenhouse effect. Changes in ozone concentrations can therefore affect atmospheric temperatures and circulation patterns. For example, ozone depletion in the stratosphere can lead to cooling in that region, which can influence weather patterns and climate variability. The interactions between ozone and climate are complex and not fully understood, but they highlight the interconnectedness of atmospheric processes and the importance of considering multiple factors when addressing environmental challenges.
- What are the potential consequences of large-scale adoption of unregulated substances on global environment? The widespread use of unregulated substances like VSLS can lead to a host of environmental problems: increased tropospheric ozone, potentially harmful to human health and agriculture; acidification, impacting sensitive ecosystems; reduced air quality, leading to respiratory problems; and disruption of atmospheric chemistry, with cascading effects on climate and pollutant transport.
- What are the specific alternatives to the ozone-depleting substances (ODS) that were phased out under the Montreal Protocol? The alternatives to ODS include Hydrochlorofluorocarbons (HCFCs), initially used as transitional replacements; Hydrofluorocarbons (HFCs), which don’t deplete ozone but are potent greenhouse gases; Ammonia and Carbon Dioxide, natural refrigerants with low environmental impact; and Hydrocarbons like propane and butane, suitable for specific applications.